I’m thrilled to share my passion and years of experience in the world of batteries with you all. You might be wondering why I’m so excited about battery capacity measurement. Well, let me tell you, it’s not just because I’m a nerd for all things battery-related, but because understanding battery capacity is crucial for making informed decisions about devices and applications that rely on batteries.
Throughout my 10+ years in the industry, I’ve seen firsthand how innovations in battery technology have impacted various sectors, from consumer electronics to electric vehicles and renewable energy storage. As someone who’s obsessed with understanding the nitty-gritty of battery performance, I can’t emphasize enough how essential it is to accurately measure battery capacity.
In this blog, I’ll be your trusty guide, sharing my knowledge and insights on battery capacity measurement techniques, standardized testing procedures, and best practices. So, buckle up and get ready to dive into the fascinating world of batteries with me!
Understanding Battery Capacity: The Heart of Power
As someone who’s been in the battery game for quite some time, I’ve grown to love and appreciate the intricacies of battery capacity. So, let’s dive right into what makes battery capacity the heartbeat of power in our lives.
Battery capacity is essentially the amount of energy a battery can store and deliver. Think of it as the battery’s “fuel tank” that powers our beloved gadgets, electric vehicles, and renewable energy systems. The larger the capacity, the more energy a battery can store and supply.
When it comes to measuring battery capacity, there are two primary units:
- Ampere-hours (Ah): This unit measures the electric charge, and is defined as the amount of current a battery can deliver for one hour. It’s like the size of a fuel tank, but for electricity!
- Watt-hours (Wh): This unit measures the energy stored in the battery, and is defined as the product of current (in amperes) and voltage (in volts) multiplied by time (in hours). Watt-hours give us a more complete picture, as they take into account the energy consumption of a device or system.
Here’s where things get really interesting. Battery capacity is influenced by a myriad of factors, making it somewhat of a moving target. Let’s break down the major players:
Factor | Effect on Battery Capacity |
---|---|
Chemistry | Different battery chemistries result in varying capacities |
Age | Capacity naturally decreases over time |
Temperature | Extreme temperatures can reduce capacity |
Discharge Rate | High discharge rates may temporarily lower capacity |
Battery capacity can be quite the enigma, but understanding these factors will help you harness the full potential of your batteries. As we move through this blog, I’ll share more of my expert insights and fun anecdotes to shed light on the incredible world of battery capacity. Stay tuned, and together, we’ll conquer the complexities of this electrifying topic!
Battery Capacity Measurement Techniques: The Art & Science
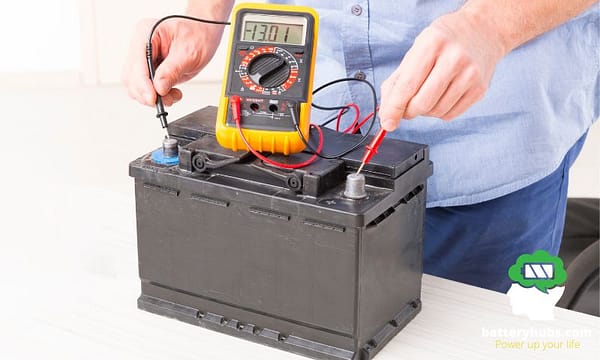
Now that we’ve grasped the basics of battery capacity, it’s time to delve into the art and science of measuring it. As a seasoned battery aficionado, I’ve experimented with various techniques and learned that there’s no one-size-fits-all approach. So, let’s explore the most popular methods, their advantages, limitations, and how they can complement each other.
Coulomb Counting: Count Your Coulombs!
Coulomb counting is a technique that directly measures the flow of electric charge (measured in Coulombs) in and out of a battery.
Advantages and Limitations
Advantages | Limitations |
---|---|
Direct charge measurement | Requires accurate current sensing |
Simple implementation | Susceptible to errors due to noise and drift |
Real-time capacity updates | Not always accurate for estimating remaining capacity |
Coulomb counting can be a useful tool, but it’s not without its quirks. For some applications, it might be just the ticket, while in others, it may fall short.
Ready to dive into the world of Coulomb counting? Let’s break down the steps for measuring battery capacity using this method and walk through a practical example.
Step-by-Step Guide to Coulomb Counting
- Choose a suitable current sensor: Select a current sensor with the appropriate range and sensitivity for your battery. Common types include shunt resistors, Hall effect sensors, and current transformers.
- Connect the current sensor: Attach the current sensor to the battery, ensuring correct polarity and a secure connection.
- Measure the current: Use a data acquisition system or a microcontroller with an analog-to-digital converter (ADC) to measure the current flowing in and out of the battery.
- Integrate the current over time: Integrate the measured current over time to obtain the total charge transfer (in Coulombs). This can be done using discrete time steps or by applying a continuous integration algorithm.
- Convert charge to capacity: Divide the total charge (in Coulombs) by 3,600 to obtain the battery capacity in ampere-hours (Ah).
Calculation Example
Let’s assume we have a battery that discharges at a constant current of 5 A for 3 hours. We want to estimate its capacity using Coulomb counting.
- Measure the current: We use a suitable current sensor and measure a constant discharge current of 5 A.
- Integrate the current over time: Since the current is constant, we can simply multiply the current (5 A) by the discharge time (3 hours) to obtain the total charge transfer:Total charge (Q) = Current (I) × Time (t) = 5 A × 3 h = 15 A·hNote that in this case, the charge is already in ampere-hours, so there’s no need to divide by 3,600.
- Determine the battery capacity: The total charge transfer is 15 A·h, which corresponds to the battery capacity.
In this example, we’ve estimated the battery capacity to be 15 Ah using Coulomb counting. Remember that this method assumes a constant discharge rate and doesn’t account for factors such as temperature or battery age, which can affect the accuracy of the measurement. However, it’s a straightforward technique that can provide valuable information about a battery’s capacity in many scenarios.
Voltage-based Methods: The Voltage Connection
Voltage-based methods rely on the relationship between a battery’s voltage and its state of charge (SOC) to estimate capacity. One common approach is to measure the open-circuit voltage (OCV) of a battery when it’s at rest.
Advantages and Limitations
Advantages | Limitations |
---|---|
Simple and non-invasive | Affected by factors like temperature and battery history |
Low-cost | Not as accurate during dynamic operating conditions |
Can provide quick estimations | Requires a stable voltage-SOC relationship |
Voltage-based methods can be a cost-effective and straightforward way to measure capacity, but they’re not without their caveats. Sometimes, a more sophisticated approach is needed.
Eager to learn about voltage-based methods for measuring battery capacity? Let’s go through the steps and explore a practical example using the open-circuit voltage (OCV) method to estimate battery capacity.
Step-by-Step Guide to Voltage-based Methods
- Create a voltage-SOC (State of Charge) curve: Obtain or create a reference curve for your specific battery type that relates open-circuit voltage (OCV) to state of charge (SOC) by performing a controlled discharge test or referring to the battery’s datasheet.
- Allow the battery to rest: Before measuring the OCV, let the battery rest for a specified period (typically 1-2 hours) to minimize the effects of voltage fluctuations due to load changes.
- Measure the open-circuit voltage: Use a digital multimeter or another accurate voltage measurement device to measure the battery’s OCV.
- Determine the SOC from the OCV: Using the voltage-SOC curve, find the corresponding SOC for the measured OCV.
- Estimate the remaining capacity: Multiply the SOC by the battery’s rated capacity to estimate the remaining capacity.
Calculation Example
Let’s assume we have a 12 V, 100 Ah lead-acid battery, and we want to estimate its remaining capacity using the OCV method.
- Create a voltage-SOC curve: We obtain the voltage-SOC curve for our lead-acid battery from the manufacturer’s datasheet. For simplicity, let’s assume the curve is linear and looks like this:OCV (V)SOC (%)12.610012.05011.60
- Allow the battery to rest: We let the battery rest for 1 hour to ensure stable OCV measurement.
- Measure the open-circuit voltage: We measure the battery’s OCV and find it to be 12.3 V.
- Determine the SOC from the OCV: Using the voltage-SOC curve, we interpolate the SOC corresponding to the measured OCV of 12.3 V:SOC = ((12.3 – 12.0) / (12.6 – 12.0)) * (100 – 50) + 50 ≈ 75%
- Estimate the remaining capacity: We multiply the SOC by the battery’s rated capacity:Remaining capacity = SOC × Rated capacity = 0.75 × 100 Ah ≈ 75 Ah
In this example, we’ve estimated the remaining battery capacity to be 75 Ah using the OCV method. Keep in mind that this method relies on a stable voltage-SOC relationship and may be less accurate under varying operating conditions or when the battery is subject to aging and temperature effects. However, it can provide a quick and non-invasive way to estimate battery capacity in many situations.
Impedance-based Methods: The Electrical Resistance Dance
Impedance-based methods, such as electrochemical impedance spectroscopy (EIS), measure a battery’s internal resistance to estimate its capacity.
Advantages and Limitations
Advantages | Limitations |
---|---|
Can provide insight into battery health | Complex and time-consuming measurements |
Sensitive to battery aging | Requires specialized equipment and expertise |
Can detect capacity fade | Not ideal for real-time, in situ capacity measurements |
While impedance-based methods offer valuable information, they may not be the most practical choice for everyday capacity measurements.
Ready to tackle impedance-based methods for measuring battery capacity? Let’s go through the steps and explore a practical example using electrochemical impedance spectroscopy (EIS) to estimate battery capacity.
Step-by-Step Guide to Impedance-based Methods
- Obtain a reference impedance-capacity curve: Obtain or create a reference curve for your specific battery type that relates impedance to capacity. This can be done by performing controlled discharge tests at different SOCs and measuring the impedance at each SOC, or by referring to the battery’s datasheet.
- Prepare the battery: Ensure the battery is at a stable temperature and in a safe condition for testing.
- Perform EIS measurement: Using specialized EIS equipment, apply a small AC voltage to the battery and measure the resulting AC current response over a range of frequencies. The impedance is calculated as the ratio of voltage to current.
- Extract the relevant impedance parameter: Analyze the EIS data to extract the relevant impedance parameter(s) that correlate with battery capacity. This might be the ohmic resistance, charge-transfer resistance, or another parameter depending on the battery type and test conditions.
- Determine the capacity from the impedance parameter: Using the reference impedance-capacity curve, find the corresponding capacity for the measured impedance parameter.
Calculation Example
Let’s assume we have a lithium-ion battery, and we want to estimate its capacity using EIS.
- Obtain a reference impedance-capacity curve: We obtain the impedance-capacity curve for our lithium-ion battery from a controlled discharge test or the manufacturer’s datasheet. For simplicity, let’s assume the curve shows a linear relationship between charge-transfer resistance (Rct) and capacity:Rct (Ω)Capacity (Ah)1010020803060
- Prepare the battery: We ensure the battery is at a stable temperature and in a safe condition for testing.
- Perform EIS measurement: We perform an EIS measurement on the battery using specialized equipment and obtain the following impedance data:Frequency (Hz)Impedance (Ω)Phase angle (°)100011-510015-251018-45
- Extract the relevant impedance parameter: From the EIS data, we identify the charge-transfer resistance (Rct) at the frequency where the phase angle is closest to -45°. In this case, Rct ≈ 18 Ω.
- Determine the capacity from the impedance parameter: Using the reference impedance-capacity curve, we interpolate the capacity corresponding to the measured Rct of 18 Ω:Capacity = ((18 – 10) / (30 – 10)) * (60 – 100) + 100 ≈ 86.7 Ah
In this example, we’ve estimated the battery capacity to be approximately 86.7 Ah using EIS. Keep in mind that impedance-based methods require specialized equipment, expertise, and more complex data analysis. They may not be suitable for real-time, in situ capacity measurements but can provide valuable insights into battery health and aging.
Hybrid Methods: The Power Combo
Hybrid methods combine two or more techniques to achieve more accurate and reliable capacity measurements.
By leveraging the strengths of different methods and compensating for their limitations, hybrid approaches can offer a more comprehensive understanding of a battery’s capacity.
Curious about hybrid methods for measuring battery capacity? Let’s go through the steps and explore a practical example that combines both coulomb counting and voltage-based methods to estimate battery capacity.
Step-by-Step Guide to Hybrid Methods
- Choose appropriate measurement techniques: Select suitable methods for your specific application, such as coulomb counting for real-time charge tracking and voltage-based methods for occasional capacity checks.
- Create a voltage-SOC curve: Obtain or create a reference curve for your specific battery type that relates open-circuit voltage (OCV) to state of charge (SOC) by performing a controlled discharge test or referring to the battery’s datasheet.
- Set up the necessary sensors and measurement equipment: Install the required sensors (e.g., current and voltage sensors) and connect them to a data acquisition system or microcontroller.
- Continuously track charge and discharge: Use coulomb counting to measure and integrate the current flowing in and out of the battery in real-time, updating the SOC as charge is transferred.
- Periodically measure OCV and adjust SOC: At specified intervals, measure the battery’s OCV, and use the voltage-SOC curve to adjust the SOC estimate derived from coulomb counting. This correction helps account for the effects of aging, temperature, and other factors on battery capacity.
- Estimate the remaining capacity: Multiply the SOC by the battery’s rated capacity to estimate the remaining capacity.
Calculation Example
Let’s assume we have a 12 V, 100 Ah lithium-ion battery, and we want to estimate its remaining capacity using a hybrid method that combines coulomb counting and voltage-based methods.
- Create a voltage-SOC curve: We obtain the voltage-SOC curve for our lithium-ion battery from the manufacturer’s datasheet. Let’s assume the curve looks like this:OCV (V)SOC (%)12.610012.05011.60
- Set up the necessary sensors and measurement equipment: We install a current sensor and a voltage sensor, connecting them to a data acquisition system or microcontroller.
- Continuously track charge and discharge: We use coulomb counting to measure the current flowing in and out of the battery. Let’s assume the battery discharges at 5 A for 1 hour, followed by charging at 3 A for 2 hours. We update the SOC as follows:Discharge: 100 Ah – (5 A × 1 h) = 95 Ah (95% SOC) Charge: 95 Ah + (3 A × 2 h) = 101 Ah (limited to 100% SOC)
- Periodically measure OCV and adjust SOC: After the charge cycle, we measure the battery’s OCV and find it to be 12.4 V. Using the voltage-SOC curve, we interpolate the SOC corresponding to the measured OCV:SOC = ((12.4 – 12.0) / (12.6 – 12.0)) * (100 – 50) + 50 ≈ 90%
- Estimate the remaining capacity: We multiply the SOC by the battery’s rated capacity:Remaining capacity = SOC × Rated capacity = 0.90 × 100 Ah = 90 Ah
In this example, we’ve estimated the remaining battery capacity to be 90 Ah using the hybrid method. By combining coulomb counting and voltage-based methods, we can achieve more accurate capacity estimation
Method | Principle | Pros | Cons |
---|---|---|---|
1. Coulomb Counting | Direct measurement of charge transfer | Real-time measurement, relatively simple setup | Affected by battery aging, temperature, and drift |
2. Voltage-based Methods | Relationship between voltage and state of charge | Non-invasive, simple measurement | Not as accurate, sensitive to temperature |
3. Impedance-based Methods | Electrochemical impedance spectroscopy (EIS) | Provides insights into battery health and aging | Requires specialized equipment, complex analysis |
4. Hybrid Methods | Combination of methods (e.g., coulomb counting and voltage-based) | Improved accuracy, accounts for various factors | More complex setup, requires multiple measurements |
Standardized Testing Procedures for Battery Capacity
Ready to dive into the world of standardized testing procedures for battery capacity? As someone with extensive experience in the battery niche, I’ve seen firsthand how crucial these tests are for consistency and comparability. Let’s embark on this electrifying journey and explore IEC 61960 and IEEE 1725 standards, the importance of standardized testing, test conditions, and interpreting test results.
IEC 61960 and IEEE 1725 standards
Two major standardized testing procedures for battery capacity are the International Electrotechnical Commission (IEC) 61960 and the Institute of Electrical and Electronics Engineers (IEEE) 1725 standards.
IEC 61960 focuses on secondary lithium-ion cells and batteries for portable applications, covering capacity, performance, and safety testing.
IEEE 1725 targets rechargeable batteries for cellular phones and includes tests for capacity, durability, and safety.
These standards ensure that battery performance metrics are consistent and reliable, enabling comparisons between different products.
Importance of standardized testing for consistency and comparability
Standardized testing is crucial for ensuring consistency in the battery industry. By adhering to a common set of test procedures and conditions, we can trust that the reported capacity and performance metrics are accurate and reliable.
Furthermore, standardized testing enables comparability between different batteries, helping consumers and professionals make informed choices based on objective data.
Test conditions (temperature, discharge rate, etc.)
A critical aspect of standardized testing is controlling the test conditions. These conditions include:
- Temperature: Tests are usually conducted at a specified temperature (e.g., 20°C) to minimize the impact of temperature fluctuations on battery performance.
- Discharge rate: Capacity tests involve discharging the battery at a specific rate (e.g., 0.2C, where C is the battery’s rated capacity) to ensure consistent comparisons.
- Charge/discharge cycles: Some tests require a specific number of charge/discharge cycles before testing to account for the battery’s initial break-in period.
These controlled conditions help minimize variability and ensure that test results are consistent and comparable across different products.
How to interpret test results
Interpreting test results involves comparing the measured capacity and performance metrics against the battery’s specifications or other batteries in the market.
Keep in mind that real-world performance may differ from test results due to factors like temperature, discharge rate, and aging. Nonetheless, standardized test results provide a valuable baseline for comparing batteries and understanding their potential performance under controlled conditions.
As the provided information covers standardized testing procedures rather than specific test results, I will create hypothetical test results for each method mentioned in the previous sections (Coulomb counting, voltage-based methods, impedance-based methods, and hybrid methods) and interpret them accordingly. Note that these examples are for illustrative purposes only.
1. Coulomb Counting Test Result
Test Result: 95 Ah Interpretation: The measured capacity of the battery using the Coulomb counting method is 95 Ah. This means that under the specific test conditions, the battery discharged a total of 95 ampere-hours before reaching its cut-off voltage. Compare this value to the rated capacity of the battery and consider the impact of real-world conditions on the battery’s performance.
2. Voltage-based Methods Test Result
Test Result: 90% State of Charge (SOC)
Interpretation: The voltage-based method test result indicates that the battery is at 90% of its rated capacity based on the measured open-circuit voltage. To estimate the remaining capacity, multiply the rated capacity by the SOC percentage. Keep in mind that voltage-based methods may be less accurate than other methods and are sensitive to factors like temperature.
3. Impedance-based Methods Test Result
Test Result: 50 mΩ
Interpretation: The impedance-based method test result shows that the battery has an internal resistance of 50 milliohms. Higher resistance values indicate increased energy loss during charging and discharging, which can affect battery performance and capacity. Comparing this value to other batteries or the manufacturer’s specifications can help determine if the battery is in good condition or if it’s experiencing performance issues due to factors like aging or temperature.
4. Hybrid Methods Test Result
Test Result: 93 Ah (Coulomb Counting) and 88% State of Charge (Voltage-based)
Interpretation: The hybrid method test result combines the Coulomb counting and voltage-based methods, yielding a measured capacity of 93 Ah and an SOC of 88%. By utilizing both methods, we can gain a more accurate understanding of the battery’s capacity and performance under the test conditions. However, remember that real-world performance may still differ due to factors like temperature and discharge rate.
These hypothetical test results serve as examples of how to interpret results from different battery capacity measurement techniques. It’s essential to compare these values to the battery’s rated capacity and consider the impact of real-world conditions on battery performance when making decisions about battery selection or maintenance.
Applications of Battery Capacity Measurement
As a battery enthusiast with years of experience, I’m excited to share some electrifying information about the various applications of battery capacity measurement. In this section, we’ll explore how capacity measurements play a vital role in consumer electronics, electric vehicles, and renewable energy storage. Let’s dive into these applications and uncover the benefits of accurately measuring battery capacity!
Consumer Electronics
Battery capacity measurements are crucial in the world of consumer electronics, from smartphones to laptops. Here are some ways capacity measurements are applied:
- Quality control for battery manufacturing: Accurate capacity measurements ensure that batteries meet required specifications, providing consistent performance and reliability.
- Battery performance comparison: By comparing capacity measurements across different batteries, consumers and manufacturers can assess performance and make informed decisions.
- Device runtime estimation: Measuring battery capacity helps manufacturers and users estimate device runtimes, providing valuable information for optimizing device usage.
Electric Vehicles
The electric vehicle (EV) industry heavily relies on accurate battery capacity measurements. Here’s how these measurements come into play:
- Range estimation: Capacity measurements enable accurate estimations of an EV’s driving range, a critical factor for consumers.
- Battery health monitoring: Regularly monitoring battery capacity helps identify potential issues, ensuring the long-term performance and health of the battery.
- Charging infrastructure planning: Accurate capacity measurements help optimize charging infrastructure planning, contributing to a more efficient and convenient EV charging experience.
Renewable Energy Storage
Battery capacity measurement is also essential for renewable energy storage systems, such as solar or wind power installations. These measurements contribute to:
- System sizing and optimization: Accurate capacity measurements help determine the optimal size and configuration of renewable energy storage systems, ensuring efficient performance.
- Battery management system (BMS) integration: Capacity measurements are crucial for BMS integration, helping monitor and manage battery performance, health, and safety.
- Performance monitoring and maintenance: Regular capacity measurements enable performance monitoring and maintenance, ensuring the long-term reliability and efficiency of renewable energy storage systems.
In conclusion, battery capacity measurements play a vital role in various applications, from consumer electronics to renewable energy storage systems. Accurate capacity measurements contribute to quality control, performance optimization, and efficient system planning, ensuring that we can continue to rely on battery-powered devices and systems for years to come.
Best Practices for Battery Capacity Measurement
As a seasoned battery aficionado, I’ve learned a thing or two about measuring battery capacity. In this section, we’ll explore some best practices for battery capacity measurement that I’ve picked up over the years. These tips will help you ensure accurate and reliable results, whether you’re a hobbyist or an industry professional. Let’s supercharge your capacity measurement skills!
Selecting Appropriate Measurement Techniques
The first step in battery capacity measurement is choosing the right technique. As we’ve discussed earlier, there are several methods available, such as Coulomb counting, voltage-based methods, impedance-based methods, and hybrid methods. It’s essential to select the method that best suits your specific application and battery type for accurate results.
Ensuring Accurate and Repeatable Measurements
Accuracy and repeatability are crucial for reliable battery capacity measurements. To ensure this, follow these best practices:
- Calibrate your equipment: Regularly calibrate your measurement tools to maintain accuracy and consistency.
- Standardize your procedures: Implement standardized testing procedures, such as IEC 61960 or IEEE 1725, to ensure consistent results across different batteries and test conditions.
- Repeat measurements: Take multiple measurements and average the results to minimize errors and improve reliability.
Considering Environmental Factors and Battery Aging
Environmental factors and battery aging can significantly impact capacity measurements. Keep these factors in mind:
- Temperature: Battery capacity can vary with temperature, so it’s essential to measure capacity at a consistent temperature or apply temperature corrections.
- Battery aging: As batteries age, their capacity decreases. Be sure to account for battery age when comparing capacity measurements.
- Discharge rate: Battery capacity can also be affected by the discharge rate. Perform measurements at a consistent discharge rate or apply corrections based on the specific battery’s performance characteristics.
Integrating Capacity Measurements with Real-World Usage Scenarios
Finally, it’s essential to integrate capacity measurements with real-world usage scenarios to make informed decisions about battery selection, maintenance, and system design. Consider the following:
- Device runtime: Use capacity measurements to estimate device runtime, taking into account factors like temperature, discharge rate, and device power consumption.
- Battery health: Regularly monitor battery capacity to assess battery health and identify potential issues that may require maintenance or replacement.
- System optimization: Use capacity measurements to optimize energy storage systems, electric vehicles, and other battery-powered devices for maximum performance and efficiency.
By following these best practices, you can ensure accurate and reliable battery capacity measurements, which are essential for informed decision-making and optimal system performance. Happy measuring!
Conclusion
And there you have it, fellow battery enthusiasts! We’ve delved deep into the world of battery capacity measurement, exploring various methods, standardized testing procedures, applications, and best practices. Armed with this knowledge, you can now confidently measure battery capacity and make well-informed decisions about battery selection, maintenance, and system design.
Remember, the key to accurate battery capacity measurement lies in selecting the appropriate method, ensuring accuracy and repeatability, considering environmental factors and battery aging, and integrating your measurements with real-world usage scenarios. By following these guidelines, you’ll be able to unlock the full potential of your batteries and optimize your battery-powered devices and systems.
As a seasoned battery expert, I’m always excited to share my knowledge and passion with others. I hope this guide has been both informative and enjoyable, sparking a newfound appreciation for the art and science of battery capacity measurement. Keep exploring, stay curious, and most importantly, have fun with your battery adventures!
As you continue to explore the fascinating world of batteries, you might be eager to learn more about their inner workings and applications. Our comprehensive resource library offers a wealth of information on everything battery-related. For instance, you can dive into the fundamentals of battery technology and understand how batteries work. You can also discover how electricity travels from a battery and the form of energy that batteries store.
For those interested in rechargeable batteries, we have detailed guides on how rechargeable batteries work, how they get recharged, and how long they last when in use. Curious about the environmental impact? We’ve got you covered with our articles on battery chemistry comparison, environmental impact of batteries, and battery disposal and recycling.
Additionally, you’ll find valuable resources on battery maintenance and care tips, battery safety, and choosing the right battery for your device. Finally, don’t forget to check out our articles on top battery manufacturers and brands and emerging battery technologies.
By leveraging our extensive knowledge base and resources, you’ll gain a deeper understanding of batteries and their applications, empowering you to make informed decisions in your personal and professional life. Happy reading!
FAQ
What are the common methods for measuring battery capacity?
The common methods for measuring battery capacity include:
- Coulomb Counting: Direct measurement of charge transfer through current integration over time.
- Voltage-based Methods: Estimation of capacity using the relationship between open-circuit voltage (OCV) and state of charge (SOC).
- Impedance-based Methods: Assessment of battery capacity through electrochemical impedance spectroscopy (EIS).
- Hybrid Methods: Combination of multiple techniques for improved accuracy.
How do you measure battery capacity in ampere-hours (Ah)?
To measure battery capacity in ampere-hours (Ah), you can use the Coulomb Counting method. Follow these steps:
- Discharge the battery at a constant current, I (amperes), and record the time, t (hours), it takes to reach the cut-off voltage.
- Calculate the battery capacity using the formula: Capacity (Ah) = I * t
What is the difference between watt-hours (Wh) and ampere-hours (Ah) in battery capacity measurement?
Watt-hours (Wh) and ampere-hours (Ah) are units used to express battery capacity:
- Watt-hours (Wh): Represents the amount of energy the battery can store and is calculated as voltage (V) multiplied by capacity in ampere-hours (Ah): Wh = V * Ah.
- Ampere-hours (Ah): Represents the amount of electric charge the battery can store, calculated as the product of discharge current (A) and time (h).
How can you use a multimeter to measure battery capacity?
A multimeter can be used to measure the open-circuit voltage (OCV) of a battery, but not its capacity directly. To estimate battery capacity using a multimeter, follow these steps:
- Measure the OCV using the multimeter’s voltage setting.
- Compare the measured voltage with the manufacturer’s voltage vs. state of charge (SOC) chart.
- Estimate the battery capacity by multiplying the rated capacity by the SOC percentage obtained from the chart.
What equipment is available for accurately testing and measuring battery capacity?
There are various equipment and instruments available for accurately testing and measuring battery capacity:
- Battery capacity testers: Devices that can perform controlled discharge tests, directly measuring capacity in ampere-hours (Ah).
- Electrochemical impedance spectroscopy (EIS) analyzers: Devices that measure battery impedance to estimate capacity.
- Battery management systems (BMS): Integrated systems that continuously monitor and estimate battery capacity using various techniques, such as Coulomb Counting or voltage-based methods.