Electricity travels from a battery by the flow of electrons along an electric field created by the difference in voltage between the two terminals. This movement of electrons powers the circuit, allowing electricity to move from the negative terminal to the positive terminal and back, completing the circuit.
I have sailed the vast seas of battery knowledge for decades and today, I’m thrilled to share with you the electrifying adventure of “How Does Electricity Travel From A Battery?”
Now, you might be thinking, “What’s the big deal? It’s just a battery!” But hold on to your hats, my friends, because batteries are the unsung heroes of our modern world, powering everything from our smartphones to electric vehicles.
In the next few paragraphs, we’ll embark on a thrilling quest to unravel the mysteries of how electricity journeys from a battery to its final destination. So, buckle up and join me, your friendly neighborhood battery expert, as we dive into this shocking tale!
Alright, adventurers, before we set sail on our electrifying voyage, let’s drop anchor and explore the foundation of our story: Battery Fundamentals. Here, we’ll learn about the magic behind batteries and how they turn chemical energy into electricity.
Battery Fundamentals
Now, I know we’re all familiar with electricity – that invisible force that powers our electronic devices and occasionally gives us a little zap! But let’s dive deeper into the essence of electricity: it’s the flow of electric charges, specifically electrons, through a material like metals. Think of it as a river of tiny particles, eagerly waiting to light up your life (or your light bulb).
Components of a Battery
Time to peek inside our mighty power source! A battery is like a tiny energy treasure chest, filled with all sorts of precious components:
- Positive Terminal
- Negative Terminal
- Electrolyte
- Positive Electrode
- Negative Electrode
Anatomy of a Battery
Now that we’ve delved into the anatomy of a battery, let’s zoom in on the first key component: the positive terminal, and explore its significance in the battery’s function.
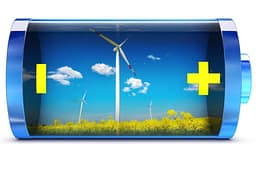
1. Positive Terminal
Ah, the positive terminal – the beacon of hope in our battery saga! This is where the positive charge gathers, eagerly waiting to join the circuit and bring light (or power) to the world.
2. Negative Terminal
Enter the negative terminal, where negative charges dwell. It’s here that our valiant electrons begin their epic journey, charging forth to conquer the realms of electrical circuits.
Internal Chemical Reactions
The heart of the battery’s magic lies within its mysterious chambers, where chemical reactions take place. These reactions convert chemical energy into electrical energy. Now, I bet you’re curious about the secret formula that ignites this alchemical transformation. Behold, the chemical reaction equation:
Anode (Negative Electrode) Reaction: Zn(s) → Zn^2+(aq) + 2e^–
Cathode (Positive Electrode) Reaction: 2MnO2(s) + H2O(l) + 2e^– → Mn2O3(s) + 2OH^–(aq)
And just like that, our battery is brimming with electric potential, ready to unleash a torrent of electrons into the waiting world of circuits and gadgets. Our electrifying journey has only just begun!
As we leave the realm of Battery Fundamentals behind, let’s embark on the most exciting leg of our journey: following the brave electrons as they navigate the treacherous seas of a battery-powered circuit. Are you ready, my fellow adventurers? Let’s set sail on the voyage of the electrons!
The Journey of Electrons
Our electrons’ epic quest begins deep within the battery, where the magic of chemistry transforms stored chemical energy into a torrent of electric charges. These daring subatomic particles are summoned by electrochemical reactions:
Anode (Negative Electrode) Reaction: Zn(s) → Zn^2+(aq) + 2e^–
Cathode (Positive Electrode) Reaction: 2MnO2(s) + H2O(l) + 2e^– → Mn2O3(s) + 2OH^–(aq)
And thus, our mighty electrons are unleashed, eager to make their mark on the world (or at least power a light bulb)!
The flow of Electrons from the Negative to the Positive Terminal
Electrons, like tiny knights in shining armor, embark on their journey from the negative terminal, where they’re brimming with energy. They valiantly charge through the external circuit, overcoming obstacles like resistors, until they reach their destination: the positive terminal.
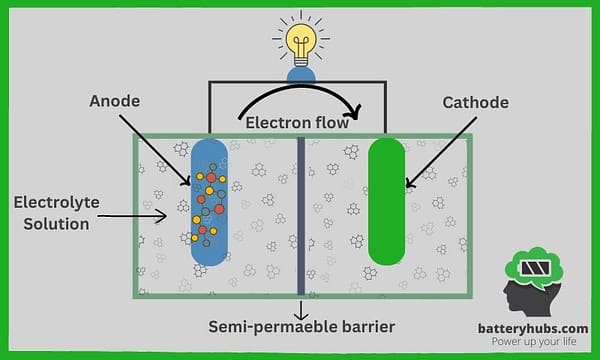
As these intrepid electrons traverse the circuit, they transfer their energy to power various devices, like a light bulb or an electric motor. Their energy gradually diminishes until they finally reach the positive terminal, ready to be recharged for another round of adventure.
Completion of an Electric Circuit
But our electrons cannot complete their quest alone! They need a trusty sidekick: the electric circuit. This closed loop of conductive material (like copper wire) forms the pathway our electrons follow, ensuring they travel from the negative to the positive terminal, powering devices along the way.
Once the circuit is connected, the electrons can flow freely, transferring their energy and creating a harmonious symphony of electrical magic!
Role of Voltage in Electric Current Flow
Voltage, my friends, is the metaphorical wind in our electrons’ sails. It’s the driving force that propels them through the circuit, like a gust of wind propelling a ship through the ocean.
In technical terms, voltage (or electric potential) represents the difference in energy between the battery’s negative and positive terminals. The greater the voltage, the stronger the force driving our electrons, and the more powerful the electric current.
And with that, our electrons have completed their grand adventure, illuminating the mysteries of battery-powered circuits for us all. Bravo, electrons! You truly are the heroes of our electrifying tale!
As our thrilling expedition continues, we shall now delve into the realm of Resistance and Ohm’s Law – the forces that govern the flow of our valiant electrons through the circuit. Ready to unravel the mysteries of resistance? Onward, my fellow explorers!
Resistance and Ohm’s Law
Resistance is the metaphorical dragon our electrons must face in their quest to power our devices. It’s the force that opposes the flow of electric current, causing our tiny heroes to slow down and expend more energy as they traverse the treacherous landscape of the circuit.
Using a Resistor to Control Electron Flow
Enter the noble resistor: a trusty companion designed to tame the mighty dragon of resistance. By adding a resistor to our circuit, we can control the flow of electrons, ensuring they don’t surge too quickly and cause havoc in our electrical kingdom.
Understanding Ohm’s Law in Relation to Battery Discharge
And now, let us pay homage to the legendary Ohm’s Law, which governs the relationship between voltage, current, and resistance in our electrifying saga:
V = I * R
In this sacred formula, V represents voltage, I denotes current, and R symbolizes resistance. The greater the resistance (R), the lower the current (I) that flows for a given voltage (V).
Imagine, if you will, our battery powering a mystical light bulb. With a voltage of 6V and a resistance of 2Ω, Ohm’s Law helps us calculate the current flowing through the circuit:
I = V / R = 6V / 2Ω = 3A
And thus, by harnessing the power of Ohm’s Law, we can safely navigate the treacherous seas of electrical circuits, ensuring our valiant electrons complete their journey and bring light to our world.
As we continue our electrifying adventure, it’s time to venture into a realm where the temperature holds sway over the flow of electric currents. Brace yourselves, dear readers, as we explore the Effects of Temperature on Electric Currents!
Effects of Temperature on Electric Currents
Temperature, the great puppeteer, can manipulate electrical resistance with its invisible strings. For instance, as the temperature rises, so does the resistance in most conductors, such as our trusty friend copper.
Imagine a copper wire carrying electricity on a sweltering summer day. As the temperature increases, the copper atoms become more agitated, causing increased resistance, and our valiant electrons face a more challenging journey through the wire.
Thermal Expansion and Contraction on Wires Carrying Electricity
But temperature’s influence doesn’t end there! It also has the power to cause thermal expansion and contraction of the wires carrying our heroic electrons. Let me paint you a vivid picture of how temperature affects wires:
In the scorching heat, the metal wires expand, causing them to stretch and become thinner. As a result, the resistance increases, and our electrons struggle to maintain their pace.
Conversely, in the frigid cold, the wires contract, becoming thicker and shorter. The resistance decreases, allowing our electrons to glide through with ease, like figure skaters on a smooth ice rink.
Ah, the mysteries of temperature and its effects on electric currents! As we continue our journey, let us keep in mind the powerful forces at play and the challenges our electrons must face as they strive to power our world. Onward, fellow explorers.
As we’ve ventured through the realm of electric currents, we’ve witnessed the might of temperature and its impact on resistance. Now, dear readers, prepare to be amazed as we delve into the world of Magnetic Fields and Energy Conservation!
Magnetic Fields and Energy Conservation
Magnetic fields are like the mystical, invisible force fields that surround an electric current. As electrons journey through a wire, they generate these magnetic fields that encircle the wire in a swirling pattern. It’s like an invisible tornado that follows our electrons as they navigate through the circuit. Now, the direction of the magnetic field depends on the direction of the electric current. If the current switches directions, so does the magnetic field. It’s like a synchronized dance between the two!
Conservation of Energy in an Electrical System
In our electrifying world, energy conservation is a principle that must not be overlooked. The energy our valiant electrons carry is conserved as it transforms from one form to another. It’s as if our electrons are frugal spenders, never wasting a single joule of energy!
Take, for example, a light bulb connected to a battery. The battery supplies the electrical energy, and our electrons carry this energy through the circuit to the light bulb. Once at the light bulb, the electrical energy is transformed into light and heat energy. The total energy remains the same, but it simply changes form. It’s a beautiful display of nature’s efficiency in action!
So, there you have it, fellow explorers! As we continue to unravel the mysteries of electric currents, let us appreciate the wonders of magnetic fields and the wisdom of energy conservation. Stay tuned for more electrifying adventures!
As we journeyed through the realms of electric currents and magnetic fields, we’ve seen the marvels of electron movement and energy conservation. Now, my dear friends, let’s apply our newfound knowledge to the real world with some Practical Tips and Safety Considerations!
Practical Tips and Safety Considerations
As we venture into practical tips and safety considerations, let me guide you through the fascinating process of how electrical energy powers devices, ensuring you’re equipped with the knowledge to use and handle batteries safely and efficiently.
How does Electrical Energy Powers Devices?
Electrical energy is what brings our devices to life. Let me walk you through the electrifying steps of how a simple battery can power a device:
- Chemical reaction: Inside the battery, a chemical reaction occurs, releasing energy and creating a flow of electrons.
- Voltage: The battery’s voltage pushes the electrons from the negative terminal to the positive terminal, creating electric potential.
- Circuit connection: When a device is connected to the battery, an electric circuit is created, allowing electrons to flow through the device.
- Electron flow: Electrons move through the circuit, carrying electrical energy to the device.
- Energy conversion: The device converts electrical energy into other forms of energy, such as light, sound, or motion.
Connecting a Battery: Which Terminal Goes First and Why
When connecting a battery, always connect the positive terminal first, followed by the negative terminal. This order is crucial to prevent short circuits, which could cause sparks, damage, or even a fire. By connecting the positive terminal first, we ensure that any accidental contact between the negative terminal and the metal chassis of a device won’t cause a short circuit.
Safety Precautions When Working with Batteries and Electrical Circuits
Safety first, my electrified companions! Working with batteries and electrical circuits can be both exciting and hazardous. Keep these safety precautions in mind to ensure a shockingly fun time:
- Disconnect power: Before working on any electrical circuit, make sure the power source is disconnected to avoid electric shock.
- Wear safety gear: Use safety goggles and insulated gloves to protect your eyes and hands from electrical sparks or shocks.
- Avoid water: Keep your work area dry, as water can conduct electricity and create dangerous conditions.
- Use proper tools: Use insulated tools designed for electrical work to minimize the risk of electric shock.
- Follow instructions: When working with batteries, always follow the manufacturer’s instructions for charging, discharging, and storage.
- Proper disposal: Dispose of used batteries properly, as they can contain harmful chemicals that are hazardous to the environment.
So, my fellow electron enthusiasts, let’s put our knowledge to good use and embark on electrifying adventures, armed with practical tips and safety precautions! Remember, safety is the key to a shockingly enjoyable experience.
Conclusion
Our electrifying journey through the world of batteries, circuits, and energy has given us valuable insights into the fascinating ways they interact. We’ve explored the basic structure of a battery, delved into how batteries work, and discovered the form of energy that batteries store. We’ve also unraveled the mystery of how a battery works in a circuit, and learned about the magic behind rechargeable batteries and how they get recharged.
We’ve discussed the importance of maintaining the charge in rechargeable batteries and evaluating how long they last when in use. Our adventure even took us deep into the inner workings of the AA battery and the origin of the term “battery”.
As we reach the end of this electrifying expedition, let us take the knowledge we’ve gained and apply it to our daily lives, whether it’s through practical tips, safety precautions, or simply satisfying our insatiable curiosity about the wonders of electricity. Remember, knowledge is power, and in this case, quite literally!
FAQ
How does a battery store energy?
A battery stores energy in the form of chemical energy. This chemical energy is stored in the electrolyte and electrode materials within the battery. When a battery is connected to an electrical circuit, a chemical reaction occurs that releases this stored energy and converts it into electrical energy, which can then be used to power electronic devices.
What is the difference between a rechargeable and a non-rechargeable battery?
A rechargeable battery is designed to be recharged multiple times after being depleted, whereas a non-rechargeable battery is designed for one-time use only. Rechargeable batteries typically use a different chemistry, which allows the chemical reactions that occur within the battery to be reversed when an external voltage is applied, recharging the battery and allowing it to be used again.
What is the role of electrical resistance in a circuit?
Electrical resistance is a property of materials that opposes the flow of electric current. It determines how easily electric charges can move through a material, and it plays a significant role in controlling the amount of current flowing through a circuit. By using resistors, you can control the flow of electrons and, in turn, adjust the performance of electrical and electronic devices.
How does temperature affect the flow of electric current in a circuit?
Temperature can have a significant impact on the flow of electric current in a circuit. Generally, as temperature increases, the electrical resistance of most materials also increases. This increased resistance can lead to a decrease in the flow of electric current. Additionally, temperature changes can cause thermal expansion and contraction of wires, which can also impact the flow of electricity.
What is the relationship between magnetic fields and electric currents?
Magnetic fields and electric currents are closely related phenomena. When an electric current flows through a conductor, such as a wire, it generates a magnetic field around the conductor. The strength and direction of the magnetic field depend on the magnitude and direction of the electric current. Conversely, a changing magnetic field can induce an electric current in a conductor.
What safety precautions should be taken when working with batteries and electrical circuits?
When working with batteries and electrical circuits, it’s essential to follow safety precautions to avoid potential hazards. These precautions include:
Always disconnect power sources before working on a circuit.
Wear insulated gloves and safety goggles to protect against electric shock.
Use insulated tools when working on electrical components.
Avoid touching live wires or components with bare hands.
Ensure proper ventilation when working with batteries, as some may release hazardous gases.
Be cautious when connecting a battery, always connecting the positive terminal first and then the negative terminal.
Familiarize yourself with the specific safety guidelines for the type of battery or electrical system you are working with.